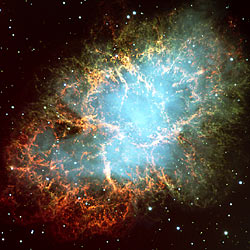
The Crab Nebula (M1) is the remnant of a supernova observed in AD 1054. Like many supernova remnants, the Crab is asymmetrical.
Courtesy European Southern Observatory.
For years theorists have used computer simulations to try to understand exactly how massive stars explode as supernovae. But they kept running into a problem: their simulated supernovae usually fizzled. Explaining how supernovae blow up so violently has remained one of the great unsolved problems of astrophysics.
Now a team led by Adam S. Burrows (University of Arizona) may have found the key missing ingredient: sound. The group, whose paper has been submitted to the Astrophysical Journal, serendipitously discovered in a recent simulation that acoustic waves generated deep in a collapsing stellar interior have the oomph to blow apart massive stars. "This could be a completely new paradigm for supernovae," says Burrows.
Theorists agree that supernovae are triggered when the core of a massive star runs out of nuclear fuel and collapses gravitationally under its own weight. The core rebounds when it reaches neutron-star density, triggering a shock wave. In the conventional view, the shock wave is energized by enormous numbers of neutrinos streaming out of the core. Even though these ghostly subatomic particles carry away more than 99 percent of a supernova's energy, they pass through matter so easily that they inject very little of their momentum into the shock wave. In most simulations, the shock wave peters out before it can blow the star to smithereens.
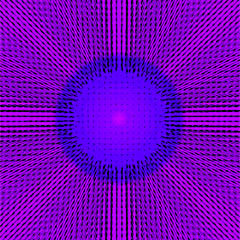
In this computer simulation frame, the core (center) of a massive star has just collapsed under the force of gravity and has rebounded, triggering an outwardly propagating shock wave (dark blue ring). At this moment, 50 milliseconds after core collapse, the center of the star remains almost perfectly symmetrical.
Courtesy Adam Burrows.
Previous groups have generally left the proto-neutron-star core out of their simulations, thinking its behavior was unimportant. But by leaving it in, and by running the simulation longer, Burrows's group found that another mechanism takes over if the neutrinos can't do the job. In short, infalling material starts hammering the core preferentially on one side. The core starts oscillating violently like a loudspeaker woofer, converting the gravitational energy of infalling material into acoustic waves that propagate outward on the opposite side of the star. The sound waves ram into one another and merge into a powerful shock wave that has sufficient energy and momentum to blow the star to Kingdom Come.
"It could be that the neutrino mechanism works for some stars and not others," says Burrows. "But if doesn't work, this acoustic mechanism can take over and explode the star."
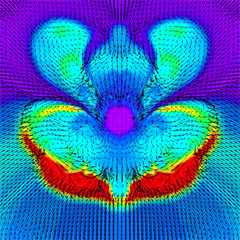
About 575 milliseconds after core collapse, the simulation shows stellar material falling onto the core at the top. This hammering process causes the core to oscillate, triggering acoustic waves (sound) that propagate outward on the bottom. The simulations show that these waves carry enough energy and momentum to blow apart the entire star. Thus a supernova is born.
Courtesy Adam Burrows.
The acoustic mechanism is highly asymmetric, giving the resulting neutron star a pronounced kick. This can explain why some pulsars race through interstellar space at speeds exceeding 1,000 kilometers per second. The simulations also show that as the acoustic-generated shock wave rips through the star's envelope, it creates conditions that enable lighter elements to stick together to form heavy elements such as gold and uranium (the so-called r-process), something that the neutrino mechanism fails to accomplish. The resulting supernova remnant is asymmetric, like most observed remnants.
An independent group led by J. Craig Wheeler (University of Texas at Austin) has also found an acoustic mechanism in recent simulations, though this model relies more heavily on the rattling of the core's magnetic field to generate sound waves. "An acoustic mechanism also emerged as a major factor in our work," says Wheeler. "I think there is something new and important going on here."
Burrows's group simulated an 11-solar-mass, non-rotating star in two dimensions. The group is now testing its model by simulating different stellar masses, adding rotation, and incorporating effects of Einstein's general theory of relativity. The ultimate test, of course, will be to compare simulation outcomes with observations.
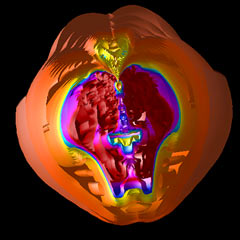
In this 3-dimensional simulation frame, a massive star explodes out the bottom, while the core of the star still accretes material from the top. The core vibrates about 300 cycles per second.
Courtesy Adam Burrows.
"It's been a long time since anything really new was introduced in this field," says theorist Stan E. Woosley (University of California, Santa Cruz), who is not a member of either the Burrows or Wheeler group. "The calculation needs to be repeated by others, it needs to be tried in different stars, and most importantly, it needs to be done in three dimensions."
Comments
You must be logged in to post a comment.