Scientists have combined observations of gravitational waves from a neutron star collision with nuclear theory to shed light on the size and nature of neutron stars.
When it comes to neutron star physics, size matters.
Neutron stars, the collapsed cores of massive stars, compress matter so tightly that atoms break apart and nearly everything converts into neutrons. As a result, about 1½ Suns’ worth of mass squeezes into these Manhattan-size objects. Most of this matter, perhaps all of it, is in the form of neutrons, but some theories suggest that deep within neutron stars, neutrons themselves dissociate, leaving a soup of quarks and gluons.
There’s no way to peer inside a neutron star, of course. But their size betrays their interior — the smaller a neutron star, the more it compresses its innards. So by taking a ruler to neutron stars, scientists can probe their nature.
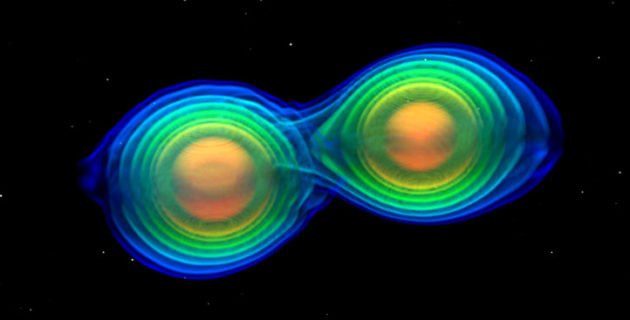
Numerical Relativity Simulation: T. Dietrich / BAM collaboration; Scientific Visualization: T. Dietrich / S. Ossokine / H. Pfeiffer / A. Buonanno
In a study appearing March 9th in Nature Astronomy, Collin Capano (Max Planck Institute for Gravitational Physics and Leibniz University Hannover, Germany) and colleagues examine the ripples in spacetime released in a neutron star merger. Combining these gravitational-wave observations with nuclear theory, the scientists estimate that a typical neutron star would span about 22 kilometers (13.7 miles)
This is the most precise measurement obtained from gravitational waves, and it has important implications for future observations.
When Neutron Stars Collide
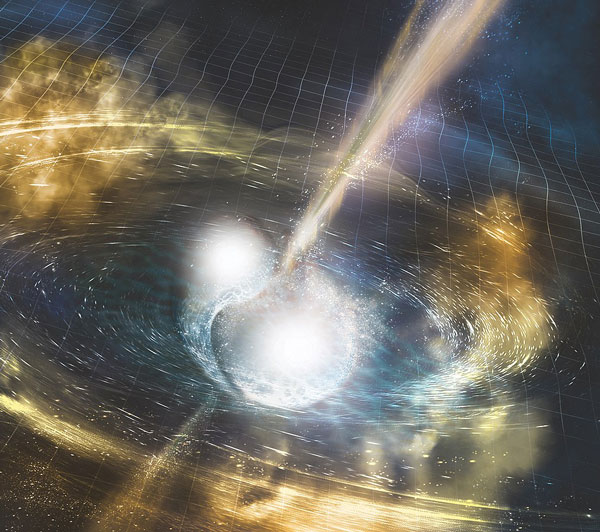
NSF / LIGO / Sonoma State University / A. Simonnet
The Laser Interferometer Gravitational-wave Observatory (LIGO) detectors witnessed spacetime ripples coming from two colliding neutron stars on August 17, 2017. The event, known as GW170817, marked the first time that astronomers could see both light and gravitational waves coming from the same source.
While the gravitational-wave signal showed the neutron stars spiraling inward and merging into a single object, light released across the electromagnetic spectrum showed the aftereffect — an explosion known as a kilonova that was visible from 130 million light-years away. At the center of the explosion, observations suggest a short-lived “hypermassive” neutron star formed that then collapsed into a black hole.
To understand the nature of the neutron stars that collided, Capano and colleagues wielded mathematics describing the nature of neutron star material, known as an equation of state. After folding in the gravitational-wave and electromagnetic observations, the equation yields the size of a typical neutron star: between 20.8 and 23.8 kilometers across. This estimate is twice as precise as previous results.
“Like we've seen in other results from analyzing GW170817, gravitational-wave astronomy has begun to meaningfully constrain the range of possibilities for neutron star matter,” says Jocelyn Read (California State University, Fullerton). “It suggests neutron stars are on the compact side.”
More compact neutron stars might mean more exotic interiors. But Read cautions that it’s not quite that simple. Even larger sizes could accommodate quark-soup cores, while smaller sizes could still be “boring” mostly-neutron objects.
Capano agrees. “We just don’t have enough data at the moment to say conclusively what happens in the core,” he says.
More gravitational-wave events will help, Capano adds, as will ongoing observations from the Neutron Star Interior Composition Explorer (NICER) instrument aboard the International Space Station. Upcoming physics experiments will also play a role in better nailing down the nuclear theory.
When a Neutron Star Hits a Black Hole
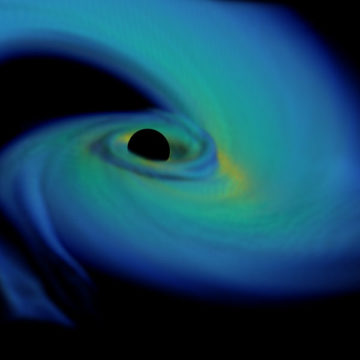
F. Foucart (U. of New Hampshire) / SXS Collaboration / Classical and Quantum Gravity, 34, 4 (2017)
Meanwhile, the compact nature of neutron stars has immediate implications for gravitational-wave observations of encounters between neutron stars and black holes. A smaller, more tightly packed neutron star would be more difficult for a black hole to tear apart before pulling it in, so black holes are more likely to swallow neutron stars whole.
That means that if a black hole and a neutron star collide, we wouldn’t expect any light-emitting counterpart — astronomers would have to rely on gravitational-wave signals alone. Only if the black hole were exceedingly small or spinning rapidly would any light be produced, Capano explains.
Nevertheless, advances in our understanding of neutron stars are on the horizon. The LIGO Collaboration, as well as the Virgo Collaboration in Italy, are analyzing results from gravitational-wave signals detected since April 2019. Their list of bona fide events, expected at the end of April 2020, should include multiple black hole mergers and at least one neutron star collision.
Comments
Anthony Barreiro
March 11, 2020 at 7:33 pm
Quark soup, rhymes with quack soup, ducks quack, Duck Soup ...
https://www.youtube.com/watch?v=Dsw9jYU_rJI
You must be logged in to post a comment.
Lou
March 12, 2020 at 7:11 am
Speaking of quark soup, I'm surprised that the latest experimental results from MIT have not been more widely reported: http://news.mit.edu/2020/force-strong-neutron-stars-0226
Yup, probably no quark stars after all...
You must be logged in to post a comment.
Monica YoungPost Author
March 12, 2020 at 1:05 pm
Fascinating! Thank you for sharing. Interestingly, the study authors found that the exact make-up of the core of the neutron star didn't affect their results much. They were using chiral effective field theory to describe the nuclear interactions. However, this theory breaks down at high densities and so scientists have to do some sort of extrapolation to describe matter in the neutron star core. What the study authors found was that the gravitational-wave data doesn't strongly favor one type of extrapolation over another. This is why Collin Capano made the statement that this research cannot constrain what happens at the center of a neutron star. We'll have to wait until we get more gravitational-wave data from neutron star collisions before we can test the theory with astronomical observations.
You must be logged in to post a comment.
Lindsay
March 13, 2020 at 8:56 pm
Why couldn't the proton and neutron maintain their identity, but a slight interpenetration of the particles be allowed such that the up and down quarks could still interact with each other: down-down and up-up repel, whereas up-down attract?
You must be logged in to post a comment.
You must be logged in to post a comment.