A single high-energy neutrino may have provided astrophysicists with telltale information about the tidal disruption of a star by a supermassive black hole some 690 million light-years away.
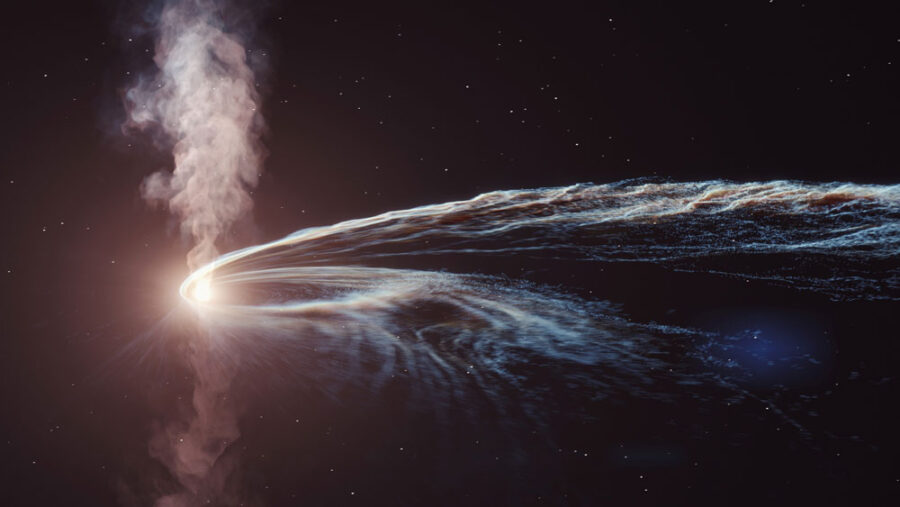
DESY / Science Communication Lab
The Neutrino and the Star-nabbing Black Hole
The sub-surface IceCube Neutrino Observatory in Antarctica detected the neutrino on October 1, 2019. The tiny, ghostlike particle was packing a punch of about 200 tera-electronvolts (15 times more energetic than what can be achieved in CERN’s Large Hadron Collider). It came from the direction of the small constellation Delphinus. While IceCube can’t precisely pinpoint the particle’s origin, the detector can narrow down the source to within an area of approximately 10 square degrees on the sky.
“Within seven hours, we were observing the area with the Zwicky Transient Facility (ZTF),” says Robert Stein (DESY, Germany). ZTF is a wide-field sky survey employing the refurbished Samuel Oschin 1.2-meter Schmidt telescope at Palomar Observatory.
Before long, Stein and his colleagues found the still-fading glow of a tidal disruption event (TDE) that had been detected on April 9, 2019, also by ZTF. These events occur when stars venture too close to a supermassive black hole; they flare as they’re torn to shreds in the strong gravitational field.
“Obviously, this was the most promising candidate” for the neutrino’s origin,” Stein says. Theorists had already suggested that TDEs might produce high-energy neutrinos, possibly in relativistic jets of plasma that shoot out from near the black hole.
The April 9th TDE, catalogued as AT2019dsg, was a rather special beast. The optical/ultraviolet outburst, which peaked in May, occurred in the nucleus of a relatively luminous galaxy, suggesting that a star had been destroyed by the tidal forces of a massive central black hole, probably weighing in at some 30 million solar masses.
“I am excited and happy that people are finding this potential association,” comments Dorothea Samtleben (Dutch National Institute for Subatomic Physics, Amsterdam), who was not involved with the find.
However, she warns that it’s not a closed case — as the researchers note, there’s still a 0.5% chance that the neutrino and the TDE have nothing to do with each other.
According to Sjoert van Velzen (Leiden Observatory, The Netherlands), who led the team that initially discovered the tidal disruption event, the 18th-magnitude source started to produce bright but rapidly fading X-rays after five weeks, while emitting increasing amounts of radio waves for many months after the initial explosion.
The radio waves result from high-energy charged particles spiraling around magnetic field lines. Apparently, the TDE is an efficient particle accelerator. “The radio data tell us that the particle acceleration process must be pretty continuous,” says Stein. “It’s not like an explosive engine,” adds van Velzen, “but more like a locomotive.”
This would explain how a TDE can produce a high-energy neutrino almost half a year after the start of the event. To create a neutrino, a high-energy proton must slam into another proton or into a photon. If particles are accelerated over a long period of time, there’s ample opportunity for neutrino production at a late stage.
How Do Black Holes Make Neutrinos?
In the February 22nd issue of Nature Astronomy, Stein, van Velzen, and 56 coauthors describe a “multi-zone” scenario for the TDE. According to this scenario, the extremely hot inner part of the transient accretion disk that forms from the torn-apart star’s remains generates X-rays close to the black hole’s event horizon. Meanwhile, at larger distances, 40,000-degree gas, also from the star, emits ultraviolet radiation. Radio waves are produced even further out, in a broad outflow. Interestingly, the researchers didn’t find any evidence for relativistic jets.
In their view, shock waves or magnetic fields accelerate the protons that are close to the central black hole. The energized protons then collide with the plentiful ultraviolet photons farther out to create high-energy neutrinos that escape the system. One of the neutrinos traveling in Earth’s direction happened to get caught in the IceCube detector some 690 million years later.
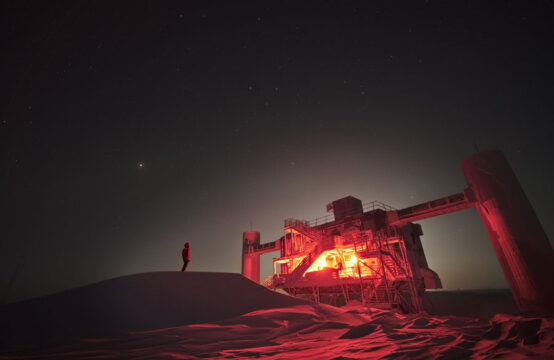
Raffaela Busse / IceCube / NSF
Writing in the same issue of Nature Astronomy, Walter Winter (DESY) and Cecilia Lunardini (Arizona State University) suggest a different scenario, partly based on the fact that the TDE’s X-ray emission faded so rapidly. Key to their scenario is the idea that the TDE did produce jets of charged particles speeding away from the black hole.
“The idea is that the [UV-producing] outflow expands and starts to cause a partial obscuration of the X-rays emitted by the accretion disk,” explains Lunardini. “The X-ray photons that are blocked by the outflow are re-emitted in all directions, and some of them end up inside the jets.” When high-velocity protons in the jets collide with those X-ray photons, they produce high-energy neutrinos.
Much remains uncertain, though. “With only one neutrino observed from a TDE, you cannot really draw firm conclusions, and the data remain open to interpretation,” admits Lunardini. Stein warns that not everyone agrees on why the X-rays disappeared so quickly. As for the existence of relativistic jets, there’s no corroborating gamma-ray evidence.
So far, the Zwicky Transient Facility has found a couple dozens of tidal disruption events, but some of their features elude explanation. “Neutrinos can help to learn more about TDEs and their diversity in the future,” Lunardini notes.
“The most important conclusion,” says Stein, “is that TDEs can accelerate particles for such a long time,” as evidenced both by the radio observations and by the late-time production of the high-energy neutrino.
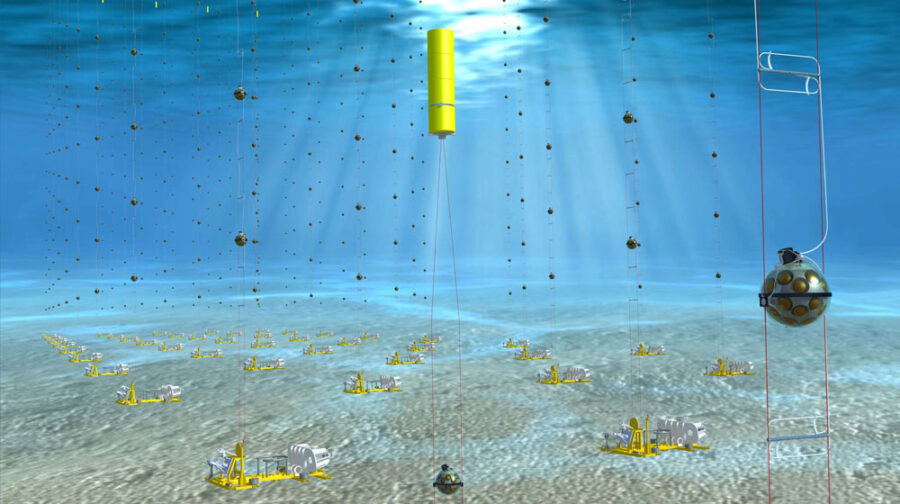
KM3NET
Samtleben, who is involved with the KM3NeT neutrino detector that is being constructed in the Mediterranean Sea, says she looks forward to a more solid link between TDEs and high-energy neutrinos. Both KM3NeT and the upcoming upgrade of IceCube could provide more examples with better pointing accuracy.
Other neutrino detectors will continue to play a role, too. Already, scientists have reported a second possible TDE-neutrino association, based on a neutrino detected in May 2020, she says.
Comments
You must be logged in to post a comment.