The absence of a mysterious X-ray signal — which could have provided evidence of dark matter — is causing as much controversy as its detection did.
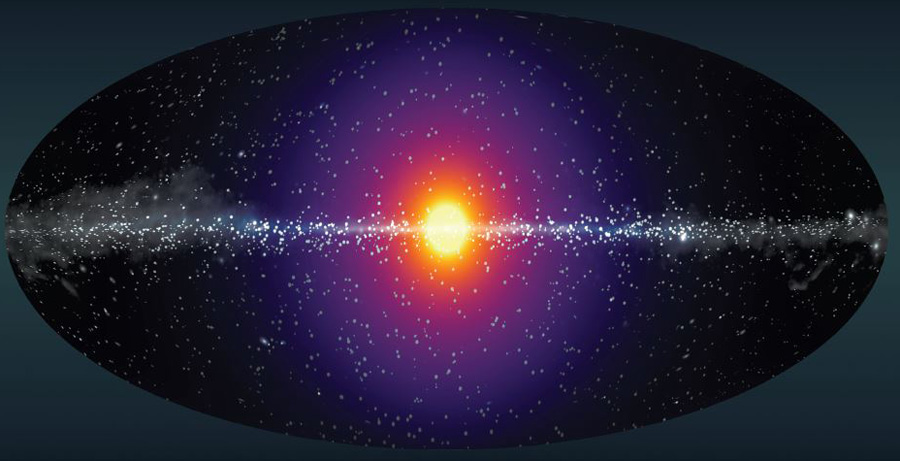
C. Dessert, N. Rodd, B. Safdi & Z. Rostomian / Fermi Large Area Telescope
Physicists have collected a year’s worth of observations of blank sky — and come up empty.
But that doesn’t mean they walked away empty-handed. The team’s “null result” could rule out one possibility for dark matter.
Christopher Dessert (University of Michigan) and his colleagues were looking for a weak X-ray signal, discovered in 2014 by Esra Bulbul (Center for Astrophysics, Harvard & Smithsonian), Alexey Boyarsky (Leiden University, The Netherlands), and colleagues. The signal has few ready explanations. First detected at the centers of clusters of galaxies, the mysterious surplus of X-rays with energies around 3,500 electron volts (3.5 keV) was later also seen in several other astronomical targets — including the Milky Way — using several different X-ray telescopes. Yet the discovery remained controversial, as observations of other targets failed to turn up the signal.
Some suspected a dark matter particle candidate — the sterile neutrino — could produce X-ray photons when it decayed; every 7 keV sterile neutrino would produce one 3.5 keV photon and one ordinary neutrino. Others thought the dark matter explanation was just bananas. Now Dessert and his fellow physicists, publishing in Science, have examined hundreds of Milky Way observations without any sign of the 3.5 keV signal. The question is, will their result hold up?
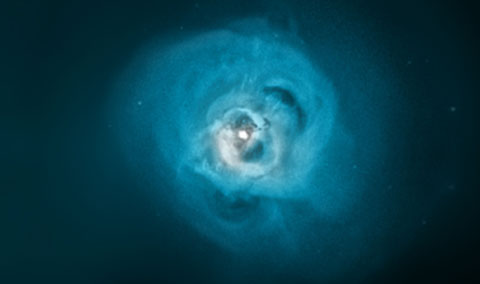
NASA / CXC / SAO / E. Bulbul & others / XMM / ESA
Staring at Blank Sky
To look for the elusive X-ray signal, Dessert and his colleagues compiled 752 observations taken with the X-ray cameras onboard the XMM-Newton satellite. They selected these observations by choosing only empty regions of the sky (blank-sky observations) containing the Milky Way halo, between 5° and 45° from the galactic center. Taken together, the observations tally up to a year’s worth of exposure time.
“It turns out that the Milky Way halo should be one of the brightest objects in the sky in dark matter decay,” says Dessert. “Although the Perseus galaxy cluster has much more dark matter, it is millions of light years away, it turns out that the signals [from Perseus and the Milky Way] would be about the same size.”
Dessert and his colleagues study the hundreds of observations simultaneously, limiting their analysis to a narrow energy range (from 3.3 to 3.8 keV). They find no sign of the 3.5 keV signal.
Furthermore, by calculating how many X-rays they should be receiving based on the dark matter model, the team is able to eliminate the 7 keV sterile neutrino as a dark matter particle candidate — if it were there, they say, they would see it. Whatever is causing the signal from distant galaxy clusters — whether it be real or contamination from background noise — it does not exist in the Milky Way’s halo.
It's All About the Background
However, this lack of signal has spurred as much controversy as the original detection. The techniques that Dessert and the other physicists have used to analyze the X-ray data run counter to astronomers’ experience.
Disentangling the X-ray signal from the background requires understanding what the background is. One source of background is real cosmic sources, whose X-rays could hide a dark matter signal.
Another source of background is “fake” X-rays produced by galactic cosmic rays and solar wind particles as they interact with the telescope and its detectors. Particles can create a signal that masquerades as a cosmic X-ray source. “For observers, particle, i.e. instrumental background, is the most challenging to estimate and eliminate,” Bulbul says.
The Milky Way observations have a very low background in the first sense, Dessert argues; that is, there aren’t a lot of cosmic X-rays interfering with the putative dark matter signal.
But Bulbul counters that the researchers haven’t contended well with the particle background. “XMM-Newton’s detector background is extremely hard to deal with because of its high variability,” Bulbul says.
Boyarsky, who like Bulbul is not involved in the current study, raises the stakes even higher. He says he has detected the 3.5 keV signal to a high degree of confidence in a subset of the very same XMM-Newton observations of the Milky Way. (His work isn’t published yet but is available on the arXiv preprint server.) Moreover, he says, there are five other published papers that have also detected the 3.5 keV signal in the Milky Way.
“The difference is that we model the background for a wide region (from 2,000 to 6,000 eV),” Boyarsky says. “Dessert and his colleagues do it for a window of about 500 eV. Why? I have no idea.” By analyzing such a narrow energy range, Boyarsky argues, Dessert and colleagues have handicapped their own analysis. “Most of the X-ray astronomers I know agree with these conclusions,” he adds.
“The statistical method we use in the paper is relatively new in X-ray astronomy, so this has led to some confusion amongst some researchers,” returns coauthor Nicholas Rodd (University of California, Berkeley. “That said, it's not a new idea. The approach is actually a robustly tested and widely used method when looking for lines in gamma-ray data or narrow features at the LHC.”
“Of course, you need an appropriate statistical method if you use a narrow energy range, which we do, that accounts for the uncertainty induced,” Rodd explains. “Our method (called profile likelihood) accounts for this.”
What's Next?
“This technique that we've developed is the most sensitive technique for probing dark matter decay to date,” Dessert says, “and we would certainly like to use it again in the future.” The physicists plan to extend their analysis to search for dark matter candidates at other energies.
When it comes to the 3.5 keV line, though, Bulbul thinks there’s not much left to be done with current instruments. “Until a new technology is in space, we will not know for certain the origin of the line,” she says. “The good news is that we are almost there.”
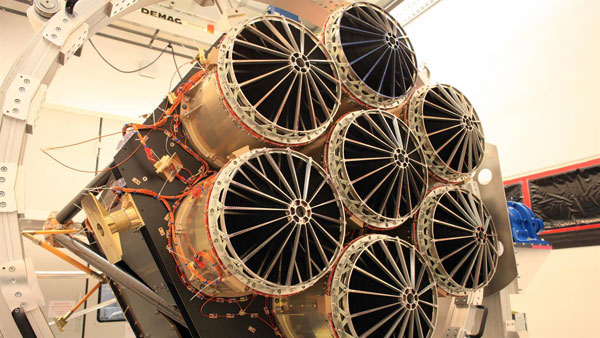
P. Friedrich / MPE
The just-launched EROSITA X-ray telescope is already collecting data on galaxy clusters, which will be used to test the existence of and origin of the 3.5 keV signal. “By the time we get our first results [with EROSITA],” Bulbul adds, “the X-ray Imaging and Spectroscopy Mission (XRISM) will be launched.” Set to fly in 2022, XRISM will carry a high-resolution calorimeter, an instrument that will give definitive measurements of the signal — and settle the controversy for good.
Comments
You must be logged in to post a comment.