The Sun flings charged particles and accompanying magnetic fields into the solar system, but how? NASA’s Parker Solar Probe dives in to find out.
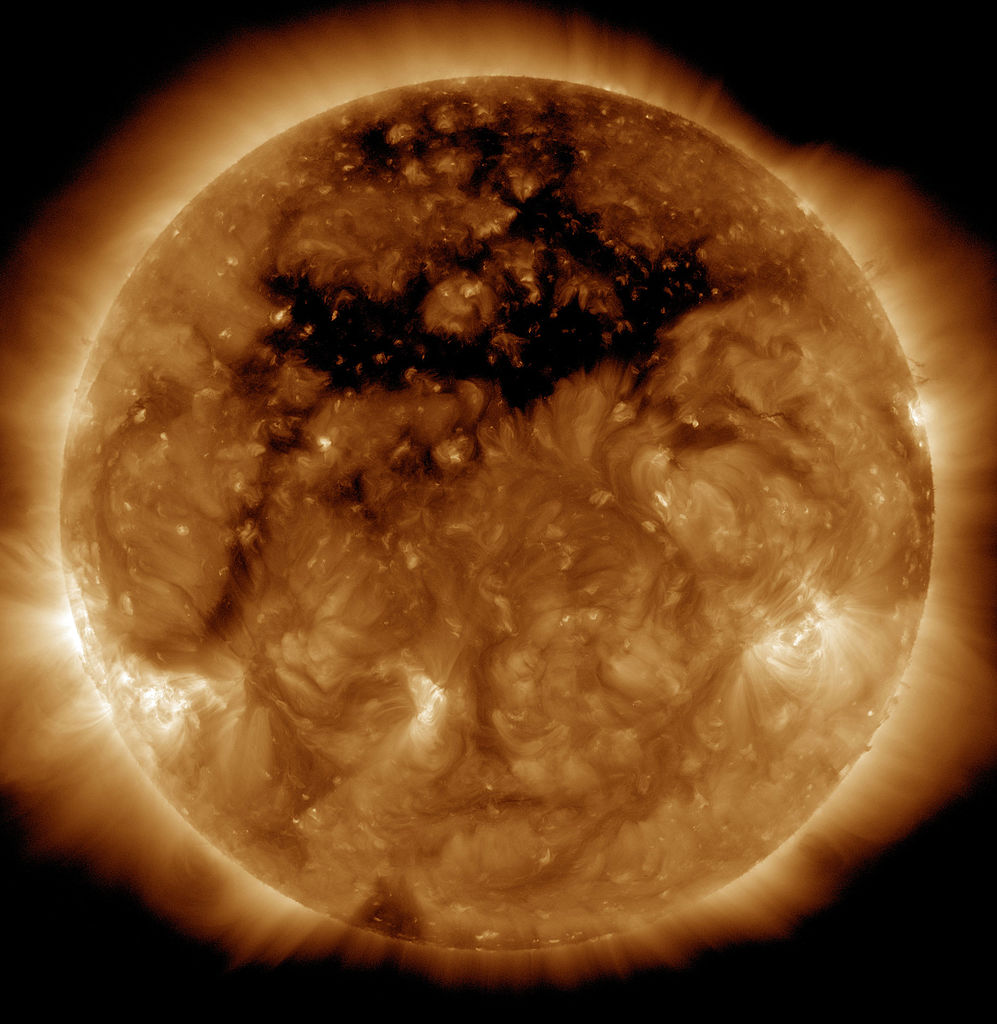
NASA / SDO
The solar wind — the flow of charged particles, or plasma, from the Sun — radiates out over all the bodies in the solar system, forming a vast, Sun-centered bubble in the dark void of space. It’s essential to life on Earth, but it also stirs up geomagnetic storms that produce power cuts, aurorae, and other earthly disturbances. The mechanism by which the solar wind arises is still not fully understood, so a team of researchers led by Stuart Bale (University of California, Berkeley) set out to tackle the quandary.
The team, whose findings are published in Nature, drew on data collected by NASA’s Parker Solar Probe. Since it launched in 2018, Parker has been circling the Sun in a series of gradually shrinking orbits that are bringing it closer to the solar surface than any previous human-made object.
Indeed, Parker has even dipped into the Sun’s atmosphere, or corona, flying as close as 5.3 million miles to the Sun’s visible surface, or photosphere, which is covered in boiling “bubbles” called granules as well as larger-scale patterns called supergranules.
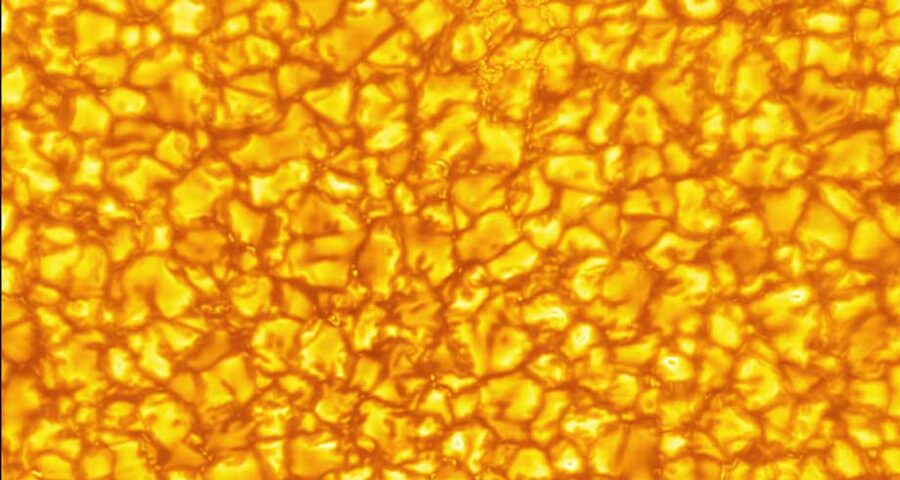
Image Credit: NSF/AURA/NSO Image Processing: Friedrich Wöger(NSO), Catherine Fischer (NSO)
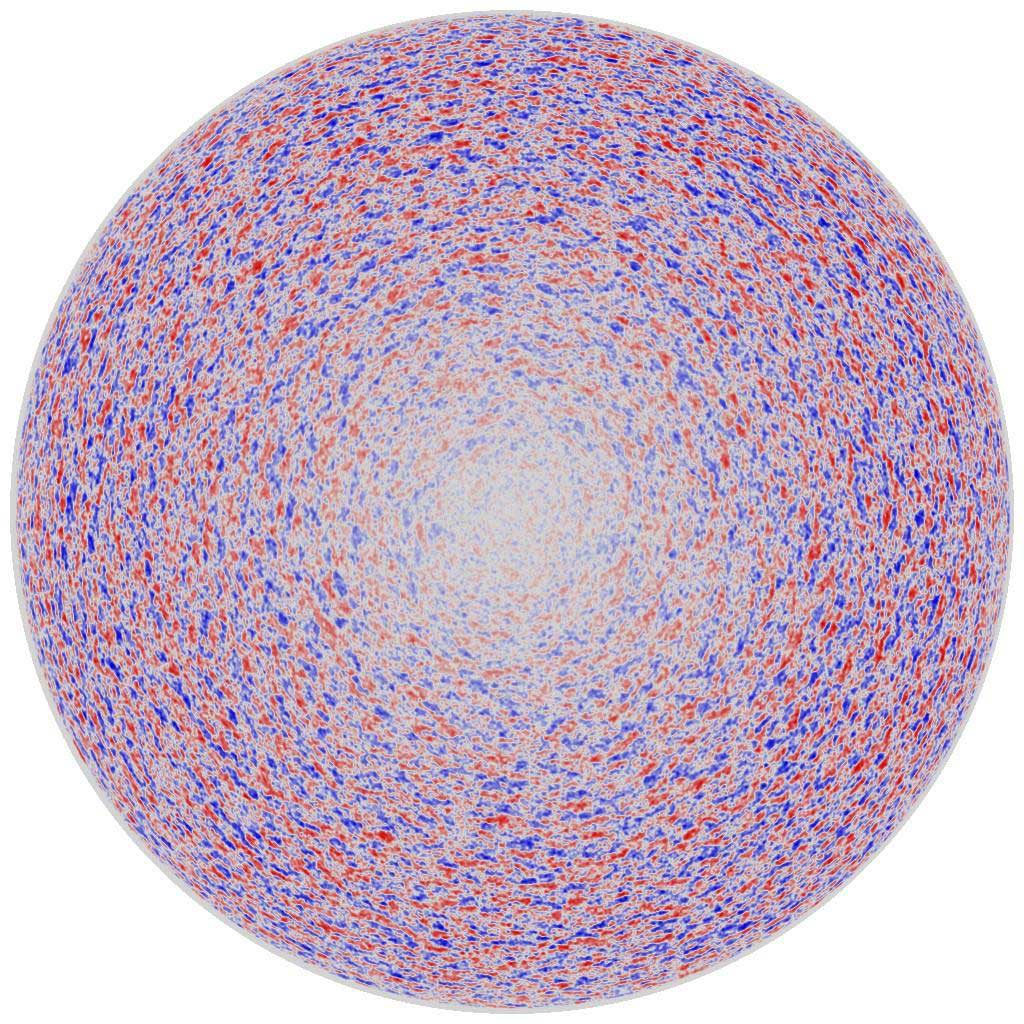
NASA Marshall Space Flight Center / D. Hathaway
Parker was deployed to answer what accelerates the plasma and accompanying magnetic fields that launch off the photosphere and into the corona. This solar wind travels at different speeds, fast and slow. While both speeds of wind are linked to magnetism in the Sun’s atmosphere, each has its own source. There’s a broad consensus that the fast solar wind originates in coronal holes, regions where the Sun’s magnetic field lines are effectively “open,” extending far into interplanetary space before looping back in.
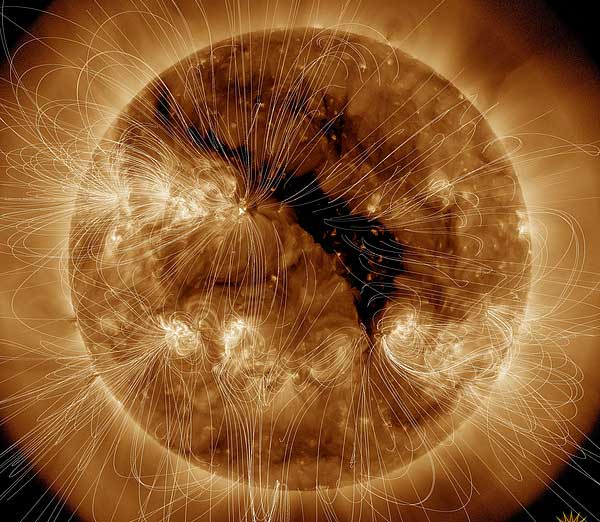
SDO/ AIA / Veronig A. & Temmer M. (University of Graz, Austria)
But what happens in these open fields to drive the solar wind? There are two competing theories. It could be either magnetic waves known as Alfvén waves, which pass through plasma, or magnetic reconnection. Both processes are known to take place on the Sun.
The researchers analyzed a range of measurements taken by Parker in November 2021, during its 10th perihelion, when it passed close to the Sun. The probe measured the solar wind’s plasma density and energy, as well as its magnetic field strength. The team combined these data with surface magnetic field measurements from the Solar Dynamics Observatory, a separate surveyor that launched in 2010.
The team saw that Parker was buffeted during its encounter by microstreams — short, jet-like bursts of high-speed solar wind. With the help of computer simulations, the team established that these microstreams are likely driven by reconnection.
According to the reconnection hypothesis, plasma is accelerated off the surface of the Sun by magnetic reconnection within the coronal holes, specifically in the regions between the supergranules.
“Where these supergranulation cells meet and go downward, they drag the magnetic field in their path into this downward kind of funnel,” Bale says. “The magnetic field becomes very intensified there because it's just jammed.”
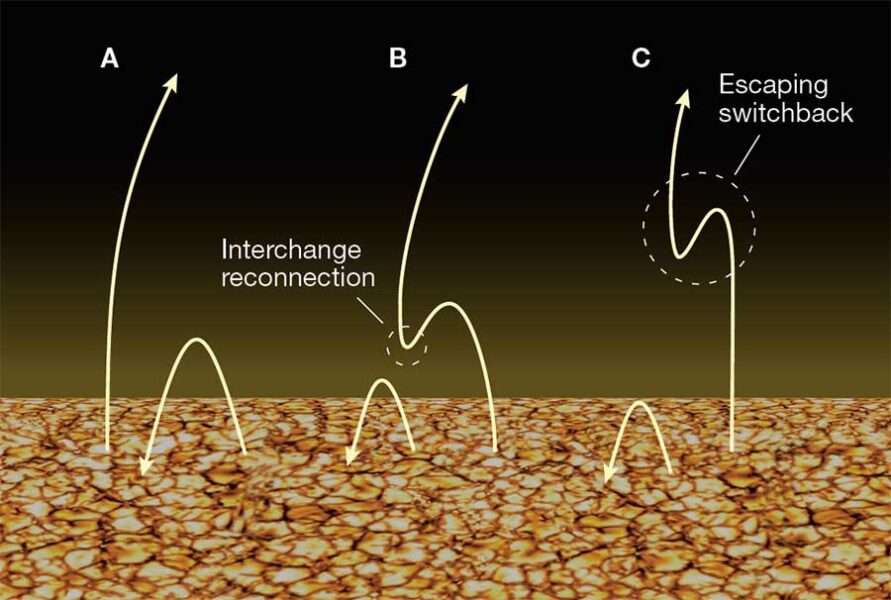
Gregg Dinderman / S&T; source: Justin Kasper / Levi Hutmacher / University of Michigan Engineering
As the funnels pull in neighboring magnetic fields, the fields reconnect, releasing magnetic energy. Microstreams of sped-up plasma are spewed out into space, along with associated sudden changes in magnetic field direction known as switchbacks. These bursts of solar wind are what hit the spacecraft.
The researchers showed this by linking the bursts and switchbacks to their footprints in two coronal holes. They also estimated the rate at which magnetic energy was released in the reconnection events and found that it was equivalent to that required to power the fast solar wind flows. In addition, they measured unusually high-energy particles in the jets, which point to the involvement of magnetic reconnection.
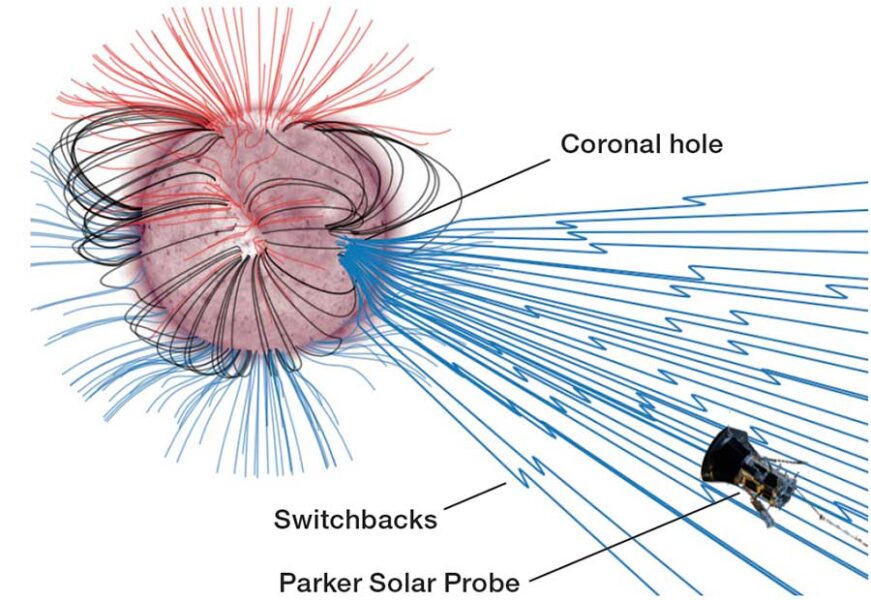
University of California, Berkeley; spacecraft image: NASA / Johns Hopkins APL
Alfvén waves, under this interpretation, are an effect of reconnection. As jets of reconnection-accelerated plasma gush out of the coronal holes, they may produce Alfvén waves. These waves may in turn boost the solar wind, but they are not the primary driver of it.
Michael Hahn (Columbia University), who wasn’t involved in the study, finds the evidence “convincing”. But understanding precisely how reconnecting magnetic fields heat and accelerate plasma remains an open question, he points out.
“The authors favor an explanation that the reconnection directly heats the plasma close to the Sun,” Hahn says. But he explains that another option mentioned in the study, indirect transfer of energy, is also possible. First, the energy goes into magnetic waves in the plasma or into turbulence, and only later, farther away from the Sun, does that energy transfer to the plasma.
The myriad energy-generating processes taking place in the corona makes it complex to identify which are the most significant, Hahn adds. This complexity presents a challenge for solar physicists to overcome.
By broadening the evidence base and bolstering the case for magnetic reconnection fueling the fast solar wind, the Nature study opens up future avenues of inquiry, such as an investigation into how much of the energy generated by reconnection is transferred into turbulence. It also paves the way for improved prediction of geomagnetic storms. Far from being the end of the matter, it represents but the latest development in an ongoing discussion.
Comments
You must be logged in to post a comment.